Investigating Heredity Beyond DNA
Why do people tend to look like their parents?
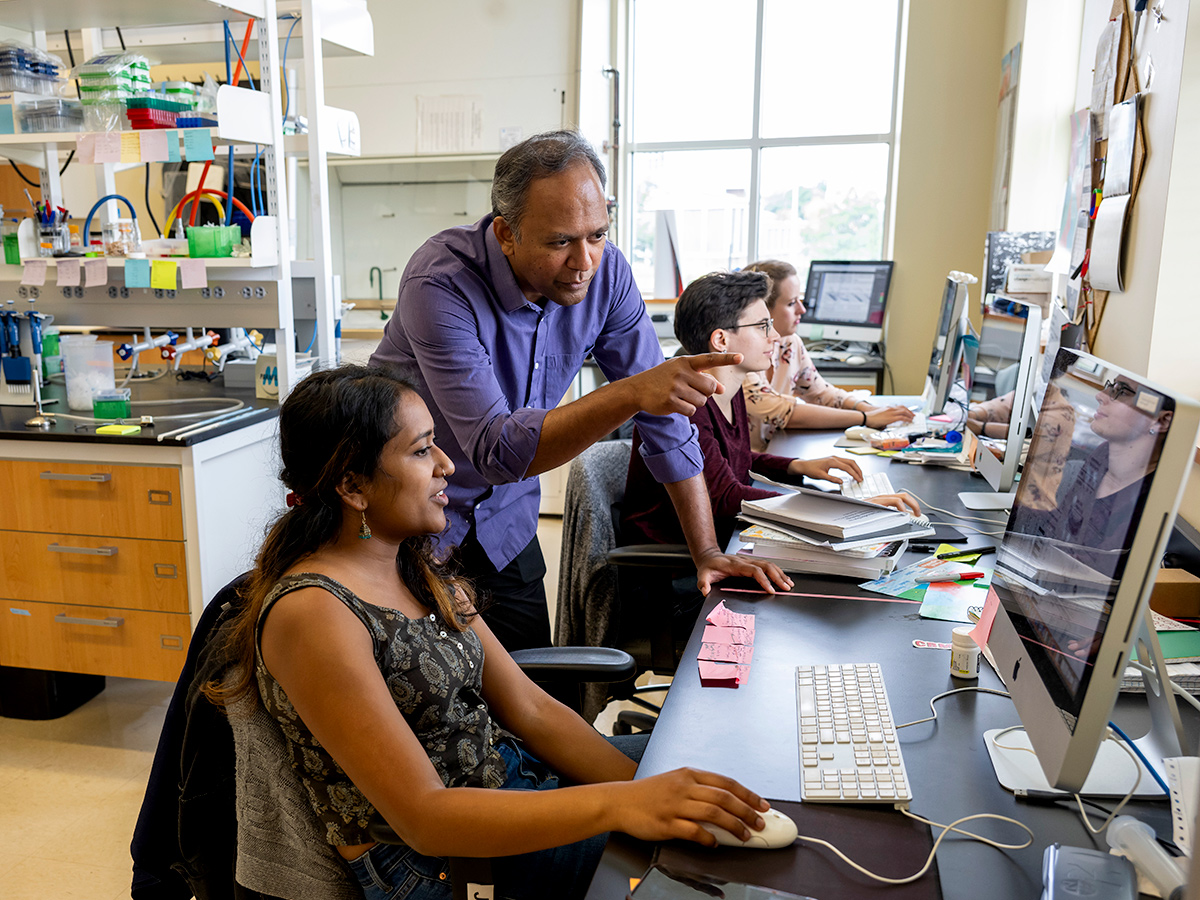
According to Antony Jose, an associate professor of cell biology and molecular genetics at the University of Maryland, the answer to this question isn’t as straightforward as it might appear. Historically, he explained, scientists only looked at genes as the basis of change in all living things, including humans. Differences between humans were associated with differences (mutations) in DNA, or deoxyribonucleic acid. For decades, scientists believed the answer to the questions of biological inheritance was in DNA, which was thought of as the ‘blueprint’ for life.
However, as modern technology and new methodologies developed over time, researchers including Jose were able to take a closer look at heredity—and discovered that DNA was not enough.
“It seems like an obvious thing that happens with all living things and their offspring, but how heredity works is far more complex than just DNA,” Jose explained. “DNA alone can’t explain how certain traits, behaviors and diseases can be transferred from one generation to the next. There has to be more to it.”
Jose compared DNA to a jumbled ‘ingredient list’ of how to build and maintain an organism, not an exact blueprint or instruction manual. While DNA contains some information about what people look like and what their offspring will look like, it does not fully explain how long a person’s hands are or why their face is shaped the way it is. Jose additionally pointed out that there is also no precise gene that fully explains how someone may have anxiety, depression or obesity.
“To explain these things, we simply need more than DNA,” he added. “The rules for making what we all share—a pair of eyes and hands, for example—are not yet known.”
Clues from RNA
These gaps in knowledge are why Jose chose to begin exploring the other major nucleic acid found in most organisms and viruses: ribonucleic acid or RNA.
In 2011, Jose started his lab at UMD to determine RNA’s role in biological inheritance. Although RNA shares some structural similarities with DNA, they ultimately perform different functions. While DNA has two strands that form the famous double helix, RNA is typically single stranded and has been most widely studied as the intermediate for making proteins using DNA sequences. But, when two RNA molecules come together to form a double helix of RNA (called dsRNA), cells recognize it as a signal to turn off genes with the corresponding DNA sequences.
Prior to arriving at UMD, Jose—as a postdoc at Harvard—used the transparent nematode Caenorhabditis elegans (more commonly known as a roundworm) as a model to show that dsRNA could circulate from an organism’s neurons to other tissues within its body. By building off that work, Jose’s lab at UMD hypothesized that the roundworm’s neuronal dsRNA could transmit the information for turning off a matching gene to all its cells. In their experiments, the researchers confirmed this guess but were surprised to discover that RNA also transmitted the information to the roundworm’s germ cells (sex cells that eventually become offspring). With that finding, Jose and his team learned that the dsRNA could ultimately ’turn off’ genes in more than 25 subsequent generations of the roundworm.
“Our findings suggested that dsRNA from a parent’s neurons could alter the expression of genes in its offspring, meaning that what happens in the present to the parent could be reflected in future descendants,” Jose explained. “Think of it this way: years ago, your parents heard some news that triggered something in their neurons. Then, those neurons sent life-changing information to the cells that would eventually become you. Because of this change in your parents’ neurons, some of your genes may never be expressed and you would not be the same person you are today.”
Jose quickly realized there were limitations to the phenomenon.
“We realized that this can’t apply to every gene. Logically, there have to be some genes that simply can’t be turned on or off forever in this way—otherwise, every living thing would have evolved very differently, and we would be very different from what we are today.”
Jose’s research extends beyond the inheritance of physical traits. In 2020, he received a Brain and Behavior Institute (BBI) Seed Grant to study how certain behaviors can be transferred or suppressed across generations. Together with Visiting Associate Professor of Biology Quentin Gaudry, Jose investigated the possibility of using synthetic biology to rewire roundworm nervous systems and engineer a particular behavior in new generations of lab-bred roundworms. Jose and Gaudry aim to build new regulatory circuits that suppress the worms’ ability to react to an odor for multiple generations.
Now, Jose and his research team are working to identify genes susceptible to silencing using RNA. Some genes, like the sequences that allow people to pass on two eyes and ears to their offspring are expected to be more stable than others, but Jose suspects certain genes are more malleable to changes brought about by an organism’s RNA through life experiences. So far, Jose has found that most genes when ‘turned off’ by RNA can return in the next generation—a fact that implies that changes in gene expression can usually dissipate or be repaired in offspring.
For Jose, it’s one more step toward unraveling the mysteries of heredity.
“All this work helps us understand where exactly nature ends and where nurture begins,” Jose stated. “Answering fundamental questions like why we look like our parents is the key to truly understanding life and why we are the way we are.”
Better ways to fight disease
Jose hopes that his lab’s work with RNA and inheritance will pave the way to a broader understanding of human diseases and better ways to treat them. He believes that RNA and the way that gene regulation is organized may help scientists understand the origins and causes of conditions that do not fully have a genetic basis like obesity, heart disease and some forms of diabetes. Although tools like CRISPR can edit certain genes known to be linked to disease, the technology has limitations.
“Right now, researchers are only dealing with the symptoms of diseases, not the root causes. Most people think that all heritable diseases can be attributed to DNA, but if there’s no single DNA mutation to pin a disease on, how do we explain why someone has the disease?” Jose said. “We don’t understand the bigger picture of gene regulation yet. But finding out how RNA can enter cells efficiently and how well it can turn on or turn off certain genes is crucial for finding and repairing conditions that are passed along without changes in DNA.”